Deteriorating Patient with Sepsis: Early Diagnosis and Intervention
Veronika Valdova • January 19, 2020
Deteriorating Patient with Sepsis: Early Diagnosis and Intervention
Published April 2017
Part of test scenarios for implementation of new sepsis guidelines
On Friday night, JB, a 23-year-old Caucasian female, presented at the emergency department with fever, chills, malaise, nausea, increasing confusion, and mild diffuse abdominal pain. Sepsis was suspected because of elevated temperature at 38.9˚C, elevated respiratory rate at 20 breaths per minute, and signs of generalized infection. The study follows the patient's condition, her development, diagnostic steps, and significant findings that led to the establishment of the diagnosis and decision which interventions to use. Full description of the patient’s condition at transfer to a non-emergency ward is described in Identification, Situation, Background, Assessment, and Recommendation forms (ISBAR) chart that is included in Appendices. In 2016, the Surviving Sepsis Campaign (SSC) prepared new revised International Guidelines for Management of Sepsis and Septic Shock (2016) (Surviving Sepsis Campaign). The progression and management of sepsis and septic shock are explained utilizing the new 2016 Surviving Sepsis Guidelines as published by Rhodes et al. (2017).
Sepsis: definition, assessment, and management
The definition of sepsis and septic shock has changed multiple times in the last three decades to reflect new research and clinical observations. As of 2016, sepsis is defined as a "life-threatening organ dysfunction caused by a dysregulated host response to infection" (Kleinpell, Schorr & Balk, 2016). The new diagnostic criteria for sepsis consist of an alteration of mental status, expressed as GCS score at 13 or below, a decrease in systolic blood pressure below 100 mm Hg, and respiration rate higher than 22 breaths per minute. A patient with two or more qSOFA criteria should be examined for organ failure (Seymour et al., 2016). The qSOFA tool is not meant to replace previously developed tools but to be used in addition to them. Systemic Inflammatory Response Syndrome (SIRS) significantly overlaps with sepsis and systemic infection (Vincent, Martin, & Levy, 2016).
The initial clinical assessment was made using the Australasian Triage Scale (ATS) approach. The Primary survey is aimed at the identification of life-threatening conditions, whilst the Secondary Survey includes a full set of vitals, comfort measures, and head-to-toe assessment (Brown, 2013). JB, a 23-year-old Caucasian female, presented at the emergency department on Friday night with fever, chills, malaise, nausea, and mild diffuse abdominal pain. The initial examination revealed heart rate at 92 bpm, SpO2 at 100%, blood pressure 140/70 mm Hg, respiratory rate 20 breaths per minute, and temperature 39.9˚C, steady regular symmetric pulse, petechial rash and adequate skin perfusion with CRT 1.5 sec. Her consciousness was assessed as 14 at the Glasgow Coma Scale (GCS) due to confusion and lethargy. The patient appeared breathless and somewhat incoherent when talking. The patient was hemodynamically stable. The condition was consistent with a generalized infection, although it did not meet the qSOFA standard for sepsis.
Early recognition of sepsis is essential for treatment success through screening. Core sets of recommendations issued by the Surviving Sepsis Campaign include routine obtaining microbiologic cultures, including blood, before starting antimicrobial therapy, providing doing so results in no substantial delay in care. Isolation of the pathogen allows the de-escalation of antimicrobial therapy (Rhodes, 2016).
Initial management of a patient with sepsis includes the stabilization of hemodynamic parameters by the administration of crystalloids and a concerted effort to identify the offending agent. The treatment of sepsis is organized into two recommendations called "bundles": the initial management that takes place within six hours after the presentation for care, and the management bundle that takes place in the intensive care unit (Dellinger et al., 2013).
The goals of the initial bundle are to lessen the immediate impact of uncontrolled infection and to assist the cardiac and respiratory systems through the use of intravenous administration of fluids and vasopressors and administration of oxygen therapy. Early diagnosis and aggressive resuscitation therapy are of critical importance — delayed or inappropriate antibiotic treatment results in higher mortality rates (Paul et al., 2010).
Once the samples for blood cultures and other parameters are collected, intravenous antibiotics shall be administered to cover all possible sources of infection. The choice of antibiotics depends on the suspected pathogen and its virulence and resistance patterns. The revised guideline recommends the administration of initial antibiotic treatment within an hour of diagnosis of sepsis or septic shock after the blood culture samples were taken. The combination of antibiotics shall then be adjusted according to the laboratory findings ("Surviving Sepsis Campaign").
Empiric broad-spectrum antimicrobial therapy is recommended to cover all likely pathogens. Antimicrobial therapy shall be revised and narrowed once the offending agent has been identified and sensitivity established, or if adequate clinical improvement occurs. The revised guideline suggests lowering of doses of antimicrobials and adjustment to the patient’s organ functions to ensure optimal pharmacokinetic and pharmacodynamics profile and decrease side effects (Rhodes, 2016). The most common bacteria isolated from patients with sepsis include Staphylococcus aureus (S. aureus), Streptococcus pyogenes (S. pyogenes), Klebsiella spp., Escherichia coli (E. coli), and Pseudomonas aeruginosa (P. aureginosa). Pathogens isolated from seriously ill patients display a wide array of virulence factors such as endotoxins, lipopolysaccharides, exotoxins and enterotoxins. Bacterial toxins modulate host cell defenses and allow the spread of infection throughout the body (Ramachandran, 2013).
JB was started on crystalloids at 30 mL/kg, a combination of two broad-spectrum antibiotics, and low flow oxygen closely monitored for any changes in hemodynamic parameters. Blood cultures, taken before the first administration of antibiotics, revealed the presence of Gram-positive cocci, eventually identified as Streptococcus pyogenes. The initial procedures were aimed at the localization of the source of infection. Ultrasound of abdomen, pelvis, and chest X-rays did not show any abnormalities. Cerebrospinal fluid was sterile. During the initial series of examinations, the patient became increasingly confused and lethargic. Chronic skin infection previously diagnosed as acne and unspecified joint pain were suspected as the most likely sources of the generalized infection. At this stage, the patient was still hemodynamically stable. The full description of the patient’s condition is in the ISBAR chart, Appendix 1.
Handover: diagnostic tests and safe parameters
Intra-hospital transfers of critically ill patients lead to increased risk of adverse events. Incidents were most likely related to equipment failure and physiological deterioration of the patient, including hypotension and hypoxia. The number of incidents can be reduced through the implementation of standardized protocols, procedures, and checklists relating to patient condition, personnel roles and responsibilities, and organization and equipment and information exchange (Brunsveld-Reinders, Arbous, Kuiper, & de Jonge, 2015). An example of such a checklist presented by Silva and Amante (2015) includes a comprehensive review of the patient’s condition before, during, and after the intra-hospital transport.
Due to multiple other medical emergencies that night, some of JB’s essential readings were not performed and recorded. The nurse who was accepting the transfer of the patient to her noticed that there were no new test results and no record of monitoring from the night before. The two most critically important measures in this particular instance were slowly progressing hypotension, significant because of a trend rather than absolute value, and hypoxemia expressed as blood lactate level. Mean arterial pressure is the critical indicator for the initiation of vasopressor treatment. Patients with mean arterial pressure (MAP) below 65 mm Hg shall be started on vasopressors such as norepinephrine and titrated up to 35-90 μg/min (Dellinger, Schorr, & Levy, 2017).
The high level of lactate is defined as > 4 mmol/L. In shock states, the elevation of lactate is caused by hypoperfusion and resulting tissue hypoxia. The functional cause of hypoperfusion is macro and microcirculatory dysfunction and mitochondrial dysfunction. The patient presentation shows a hypermetabolic state (Andersen et al., 2013). Blood lactate levels in septic patients are the indicator of cellular metabolic failure and low tissue oxygenation rather than global hypoperfusion. Lactate is the most important indicator in patients with sepsis because of its implications on treatment decisions. All patients with elevated lactate > 4 mmol/L (36 mg/dL) shall be treated for septic shock regardless of blood pressure (Dellinger, Schorr, & Levy, 2017).
The rationale for transfer to a non-emergency ward was that the patient no longer met the MET criteria while on medication that could be administered elsewhere. Her normalizing body temperature at 37˚C was misinterpreted as a sign of recovery from the infection. Her blood pressure was 90/60 mm Hg, respiratory rate at 18, and she was˚ alert. Ominous trends that would suggest otherwise were not available. Accurate and current clinical assessment is essential for a clinician to be able to make a decision whether or not the patient can be transferred to non-emergency care. In JB’s case, the patient’s dropping temperature was misinterpreted as improvement and a sign of a clearing infection rather than a symptom of developing sepsis. However, it would be incorrect to blame a nurse at ICU for failure that is of organizational nature. Comprehensive checklists and protocols relevant to patient transfer would substantially improve patient safety.
Septic shock: pathophysiology and assessment
“Septic shock is a subset of sepsis in which circulatory, cellular, and metabolic alterations are associated with a higher mortality rate than sepsis alone” (“Surviving Sepsis Campaign”). Septic shock is the result of the maldistribution of blood flow. Hypo-perfusion of tissues leads to tissue hypoxia, metabolic acidosis, the release of cytokines and oxygen free radicals, and cell death. The dysfunction of multiple organ systems is quickly followed by multiple organ failure if the process is not halted. Systemic Inflammatory Response Syndrome (SIRS) is characterized by elevated or lowered white blood cell count or an increase in immature neutrophils (bands). Resulting progressive sepsis-related organ dysfunction is called Multiple Organ Dysfunction Syndrome (MODS) (Kleinpell, Schorr & Balk, 2016).
Septic shock is the most critical stage of sepsis and results in the highest mortality rates. When the infection is cleared, and tissue recovers, organ injury and secondary infections may occur. It is important to understand that sepsis involves inflammatory, hemodynamic, tissue-perfusion, and organ dysfunction variables, with the potential to advance to organ dysfunction. The complexity of sepsis and the possible deterioration of septic shock are dependent on the virulence and load of the causative pathogen and the present state of health and genetics of the host. Pro-inflammatory reactions that attack the pathogen are responsible for parallel tissue damage. Anti-inflammatory responses limit tissue injury, but also promote susceptibility to secondary infection (Angus, & van der Poll, 2013).
Septic shock develops in patients who remain hypotensive despite the administration of vasopressors and whose serum lactate levels increase above 2 mmol/L despite adequate fluid resuscitation. Tissue hypo-perfusion results in hypoxia, which triggers a cascade of biochemical processes within the cell due to anaerobic metabolism and the resulting production of lactate. Release of cytokines, namely tumor necrosis factor-alpha (TNF-α), interleukin-1 (IL-1), and other pro-inflammatory mediators, leads to increased permeability of membranes and leakage of intravascular fluid into interstitial space. Some bacterial toxins activate the same cascade. Activation of interleukins 6 and 8 (IL-6 and IL-8) leads to the release of platelet-activating factor and formation of micro-thrombi within the vascular system. The combination of these factors results in the impaired distribution of blood volume, vasodilatation, and hypoperfusion — resulting in tissue hypoxia, which further exacerbates and worsens the problem. Activation of the central nervous system and hypophyseal-adrenocortical axis leads to hypermetabolic state, increasing tissue demand for oxygen. The effect of TNF-α and IL-1 on the myocardium is the cause of depressed myocardial function, decreased ejection volume, and worsening hypotension. Hypovolemia leads to centralization of blood circulation, limiting the flow of blood in the kidneys and mesenterium, causing additional complications such as acute kidney injury (Brown, 2013).
Indicators of septic shock include persisting hypotension below 65 mm Hg despite vasopressors use, and blood lactate > 2 mmoL/L despite adequate volume resuscitation (Seymour et al., 2016). Lactate is the product of anaerobic metabolism, produced by most cells in the human body. Under anaerobic conditions, it is the end-product of glucose metabolism. Under normal conditions, lactate is rapidly cleared from the bloodstream by the liver. Elevated lactate is defined as 2 to 2.5 mmol/L by different sources. The high level of lactate is defined as > 4 mmol/L. The term lactic acidosis shall be used if the patient has high lactate and pH<7.35. In shock states, the elevation of lactate is caused by hypoperfusion due to macro and microcirculatory dysfunction, mitochondrial dysfunction, and the presence of a hypermetabolic state (Andersen et al., 2013). Blood lactate levels in septic patients are the indicator of cellular metabolic failure and low tissue oxygenation rather than global hypoperfusion. Although lactate does not provide a full picture of metabolic status as blood gases do, it is considered as the most important indicator in patients with sepsis because of its implications on treatment decisions. All patients with elevated lactate > 4 mmol/L (36 mg/dL) shall be treated for septic shock regardless of blood pressure (Dellinger, Schorr, & Levy, 2017).
Accurate and rapid assessment is vital to halt the progression of the sepsis into septic shock. The patient may or may not present with symptoms of bacteremia with or without complications such as acute respiratory distress syndrome (ARDS), acute kidney injury (AKI), disseminated intravascular coagulation (DIC), myocardial ischemia and dysfunction, mesenteric ischemia and other complications relating to hypo-perfusion and organ dysfunction (Kalil and Bailey, 2016).
Invasive monitoring of patient conditions in septic shock is possible through the use of transpulmonary thermodilution (Saugel et al., 2016). The technique is performed through the insertion of a central venous catheter into the inferior or superior vena cava; a thermistor-tipped arterial catheter is usually placed through the femoral artery into the abdominal aorta (Bendjelid, Giraud, Siegenthaler, & Michard, 2010). Cooled saline is introduced into the central venous circulation and passes through the right heart, pulmonary circulation, and the left heart. Then the thermistor detects the thermal indicator bolus of sale at the end of the arterial catheter, allowing the realization of a curve that shows how the cold indicator is diluted through the patient’s cardiopulmonary circulation. Other hemodynamic values available are CO assessment, cardiac preload, myocardial contractility, and the extravascular lung water index (Kiefer et al., 2012; Tagami et al., 2010 and Casserly, Read, & Levy, 2011).
After transfer, JB’s GCS was still 13; her blood pressure dropped to 90/60 mm Hg heart rate increased to 141 bpm, and the temperature dropped to 37˚C and continued to drop. Her pulse was weak and narrow, and respiratory rate > 22 breaths per minute. Peripheral capillary oxygen saturation (SpO2) dropped to 90%. Her skin turned very pale and showed signs of hypo-perfusion and edema. Laboratory findings included leukocytosis, neutrophilia with a left shift, and hyperglycemia. JB’s qSOFA score increased from one on the Friday night to three by Sunday mid-day when she developed tachypnea and systolic hypotension in addition to the Glasgow Coma Scale score (GCS) of 13. JB’s condition indicated that she is developing septic shock. She was hypotensive, and her mean arterial pressure (MAP) was dropping, and her lactate level was 3.5 mmol/L. By Sunday night, JB was unable to void despite high fluid intake. Oliguria increased creatinine and blood urea nitrogen, and electrolyte imbalance pointed to kidney dysfunction. Moreover, JB started showing signs of dyspnea. Using the Berlin Definition, her ADRS was 200 mm Hg and PaO2/FIO2 ≤ 300 mm Hg, which qualifies as mild (Ranieri, Rubenfeld, Thompson, & et al., 2012).
Septic shock: management
It is essential to ensure good perfusion and oxygenation of tissues. It is key to halt the deterioration of a patient with sepsis into septic shock and prevent additional tissue injury. A patient with sepsis-induced hypotension or lactate above 4 mmol/L shall receive a rapid infusion of crystalloids and low flow oxygen. Patients with pneumonia or acute lung-injury may require high flow oxygen and intubation or mechanical ventilation. When large volumes of crystalloids are required, supplementation of albumin fluid should be considered to maintain intravascular volume. The septic shock is characterized by hypotension that has not responded to previous efforts at fluid resuscitation. The first consideration is, therefore, the use of vasopressors. The current vasopressor of choice is norepinephrine to maintain a MAP of ≥65 mmHg, followed by vasopressin, epinephrine, and phenylephrine (Rhodes et al., 2016 and Pittman, 2016)
Patients with mean arterial pressure (MAP) below 65 mm Hg shall be started on vasopressors such as norepinephrine and titrated up to 35-90 μg/min. Once stabilized, norepinephrine can be continued alone or in combination with vasopressin at 0.03 units/min, and the dose of norepinephrine can be gradually decreased. If the patient continues to deteriorate, epinephrine and phenylephrine can be added to achieve the MAP target (Dellinger, Schorr, & Levy, 2017). Care must be taken to avoid excessive fluid resuscitation, as a negative fluid balance is preferred with sepsis patients; particularly in patients with ARDS, negative fluid balance improves organ function (Saugel et al., 2016).
The new guidelines suggest the use of dobutamine only patients with low risk of tachycardia and only in low doses for renal protection. Attempts to increase cardiac output with dobutamine did not improve outcomes. Dobutamine, and to a limited extent some other inotropes, may improve tissue perfusion through increasing oxygen delivery (Rhodes et al., 2016). Recommended interventions include prophylaxis of gastric ulcers and venous thromboembolism and insulin for glucose control. Other interventions, such as sedation or dialysis, are reserved for special situations. It is of critical importance to locate the source of infection. Corticosteroids, immunoglobulins, blood purification, administration of blood products, bicarbonates, parenteral nutrition, and inotropes are not recommended in patients with septic shock (Rhodes, 2017).
JB did not initially respond to norepinephrine but eventually stabilized when vasopressin and epinephrine were added to the infusion. Oxygen was administered per nasal cannula. Antibiotic treatment was adjusted to kidney function and to target Gram-positive cocci. Because of a large volume of crystalloids and continuing extravasation, she received albumin to maintain intravascular volume. A central venous catheter capable of hemodynamic measuring was inserted to enable peripheral access and administration of intravenous fluids. To normalize her blood glucose levels, she received insulin.
Conclusion
Sepsis is a serious condition that still has a high mortality despite advanced care. The key to successful treatment is early diagnosis and aggressive treatment. Recently revised treatment guideline offers an excellent opportunity to review hospital practices in regards to the diagnosis and early aggressive treatment of sepsis and septic shock. Screening for infections, including blood cultures, facilitates early diagnosis and enables timely intervention. The case scenario presents a relatively low-risk patient demographic presented with symptoms that could have been easily misdiagnosed. The clinical symptoms corresponded with sepsis and rapidly progressed into septic shock. The patient benefited from early diagnosis and aggressive intervention based on newly revised guidelines, and the fact that these guidelines were implemented in clinical practice with fidelity. However, the patient later deteriorated during a poorly controlled intra-hospital transfer that was signed off by a nurse who used a standard that should not have been applied in this situation, consequently causing a delay in the detection of deterioration and delayed care. Whilst the case shows evidence-based medicine at its best, more can be done at the organizational level to facilitate communication during transfers, hospital information management during handovers, including the design of checklists and application of appropriate standards as well as roles and responsibilities for standard situations.
References
Andersen, L., Mackenhauer, J., Roberts, J., Berg, K., Cocchi, M., & Donnino, M. (2013). Etiology and Therapeutic Approach to Elevated Lactate Levels. Mayo Clinic Proceedings, 88(10), 1127-1140. http://dx.doi.org/10.1016/j.mayocp.2013.06.012
Angus, D., & van der Poll, T. (2013). Severe Sepsis and Septic Shock. New England Journal of Medicine, 369(9), 840-851. http://dx.doi.org/10.1056/nejmra1208623
Bendjelid, K., Giraud, R., Siegenthaler, N., & Michard, F. (2010). Validation of a new transpulmonary thermodilution system to assess global end-diastolic volume and extra- vascular lung water. Critical Care, 14(6), R209. http://dx.doi.org/10.1186/cc9332
Brown, D. (2013). Lewis's Medical Surgical Nursing (1st ed., pp. 1706-1729). Elsevier Health Sciences APAC.
Brunsveld-Reinders, A., Arbous, M., Kuiper, S., & de Jonge, E. (2015). A comprehensive method to develop a checklist to increase safety of intra-hospital transport of critically ill patients. Critical Care, 19(1). http://dx.doi.org/10.1186/s13054-015-0938-1
Casserly, B., Read, R., & Levy, M. (2011). Hemodynamic Monitoring in Sepsis. Critical Care Nursing Clinics Of North America, 23(1), 149-169. http://dx.doi.org/10.1016/j.ccell.2010.12.009
Dellinger, R., Levy, M., Rhodes, A., Annane, D., Gerlach, H., & Opal, S. et al. (2013). Surviving sepsis campaign: international guidelines for management of severe sepsis and septic shock: 2012. Crit Care Med., 41(2), 580-637. http://dx.doi.org/10.1097/CCM.0b013e31827e83af.
Dellinger, R., Schorr, C., & Levy, M. (2017). A users’ guide to the 2016 Surviving Sepsis Guidelines. Intensive Care Medicine, 43(3), 299-303. http://dx.doi.org/10.1007/s00134-017-4681-8
Kalil, Andre, and Kristina L Bailey. "Septic Shock". Medscape (2016): n. pag. Web. 10 Apr. 2017.
Kiefer, N., Hofer, C., Marx, G., Geisen, M., Giraud, R., & Siegenthaler, N. et al. (2012). Clinical validation of a new thermodilution system for the assessment of cardiac output and volumetric parameters. Critical Care, 16(3), R98. http://dx.doi.org/10.1186/cc11366
Kleinpell, R., Schorr, C., & Balk, R. (2016). The New Sepsis Definitions: Implications for Critical Care Practitioners. American Journal Of Critical Care, 25(5), 457-464. http://dx.doi.org/10.4037/ajcc2016574
Paul, M., Shani, V., Muchtar, E., Kariv, G., Robenshtok, E., & Leibovici, L. (2010). Systematic Review and Meta-Analysis of the Efficacy of Appropriate Empiric Antibiotic Therapy for Sepsis. Antimicrobial Agents and Chemotherapy, 54(11), 4851-4863. http://dx.doi.org/10.1128/aac.00627-10
Pittman, R. (2016). Regulation of Tissue Oxygenation, Second Edition (1st ed.). San Rafael: Biota Publishing.
Ramachandran, G. (2013). Gram-positive and gram-negative bacterial toxins in sepsis. Virulence, 5(1), 213-218. http://dx.doi.org/10.4161/viru.27024
Ranieri, V., Rubenfeld, G., Thompson, B., & et al. (2012). Acute respiratory distress syndrome: the Berlin Definition. JAMA, 307, 2526-2533. http://dx.doi.org/10.1001/jama.2012.5669
Rhodes, A., Evans, L., Alhazzani, W., Levy, M., Antonelli, M., & Ferrer, R. et al. (2017). Surviving Sepsis Campaign. Critical Care Medicine, 45(3), 486-552. http://dx.doi.org/10.1097/ccm.0000000000002255
Saugel, Bernd et al. "Advanced Hemodynamic Management In Patients With Septic Shock". BioMed Research International 2016 (2016): 1-11. Web.
Silva, R., & Amante, L. (2015). Checklist for the intrahospital transport of patients admitted to the Intensive Care Unit. Texto & Contexto - Enfermagem, 24(2), 539-547. http://dx.doi.org/10.1590/0104-07072015001772014
Seymour, C., Liu, V., Iwashyna, T., Brunkhorst, F., Rea, T., & Scherag, A. et al. (2016). Assessment of Clinical Criteria for Sepsis. JAMA, 315(8), 762. http://dx.doi.org/10.1001/jama.2016.0288
Surviving Sepsis Campaign. (2017). Survivingsepsis.org. Retrieved 8 April 2017, from http://www.survivingsepsis.org/About-SSC/Pages/default.aspx
Tagami, T., Kushimoto, S., Yamamoto, Y., Atsumi, T., Tosa, R., & Matsuda, K. et al. (2010). Validation of extravascular lung water measurement by single transpulmonary thermodilution: human autopsy study. Critical Care, 14(5), R162. http://dx.doi.org/10.1186/cc9250
Vincent, J., Martin, G., & Levy, M. (2016). qSOFA does not replace SIRS in the definition of sepsis. Critical Care, 20(1). http://dx.doi.org/10.1186/s13054-016-1389-z
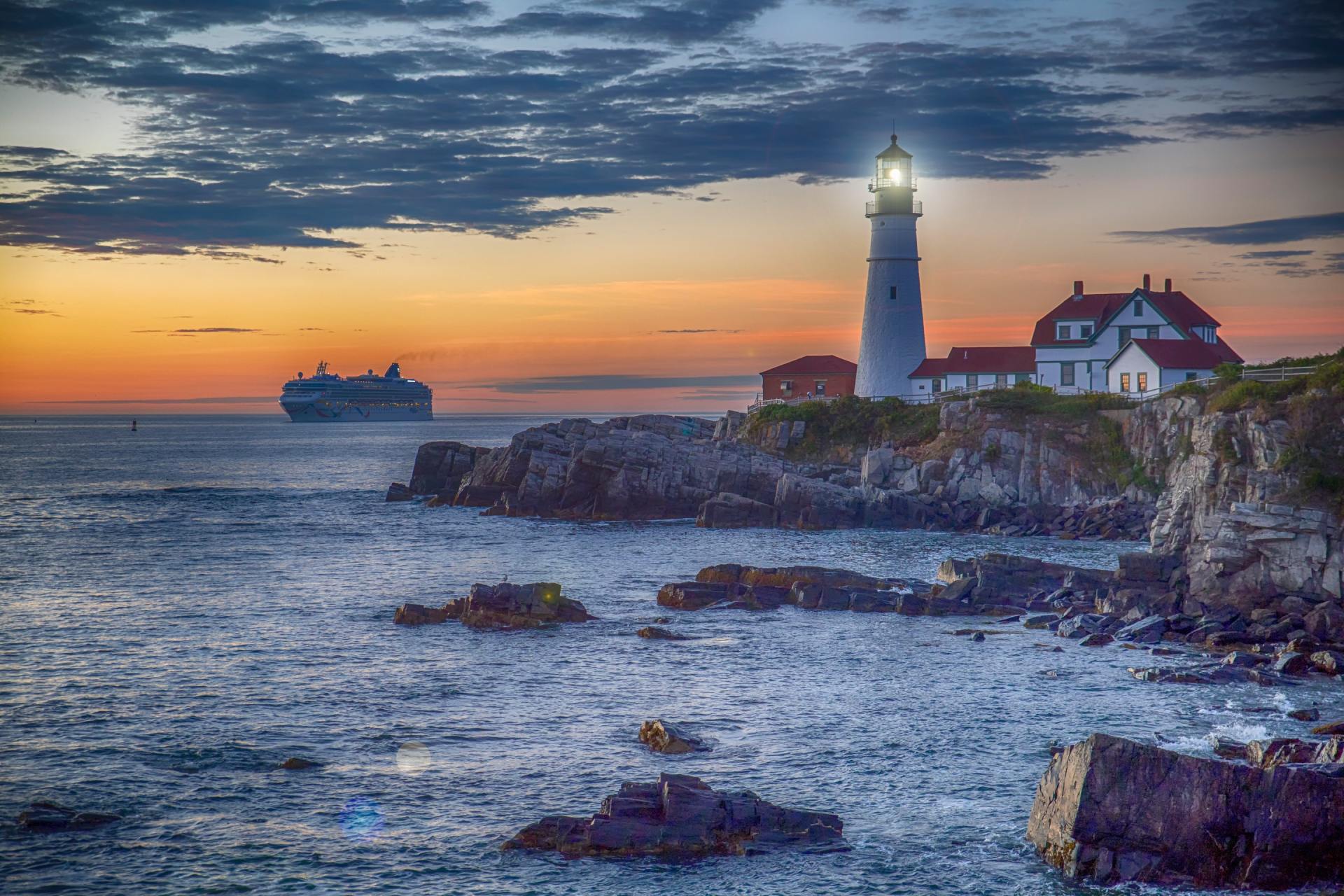
Nařízení (EU) 2017/745 o zdravotnických prostředcích vstoupilo v platnost 26. května 2021. Platnost certifikátů vydaných podle stávajících směrnic vyprší nejpozději 24. května. Toto je třeba provést ve lhůtě, která odpovídá době nezbytné pro přezkoumání dokumentace a před vypršením platnosti současných certifikací. Česká republika stále nemá svůj oznámený subjekt. Jakmile dojde k akreditaci, bude ITC zavalen ohromným počtem nevyřízených podání v českém jazyce. Zajisdtěte si pomoc s podáním od týmu Arete-Zoe, který má s přípravou klinické dokumentace dle MDR bohaté zkušenosti.
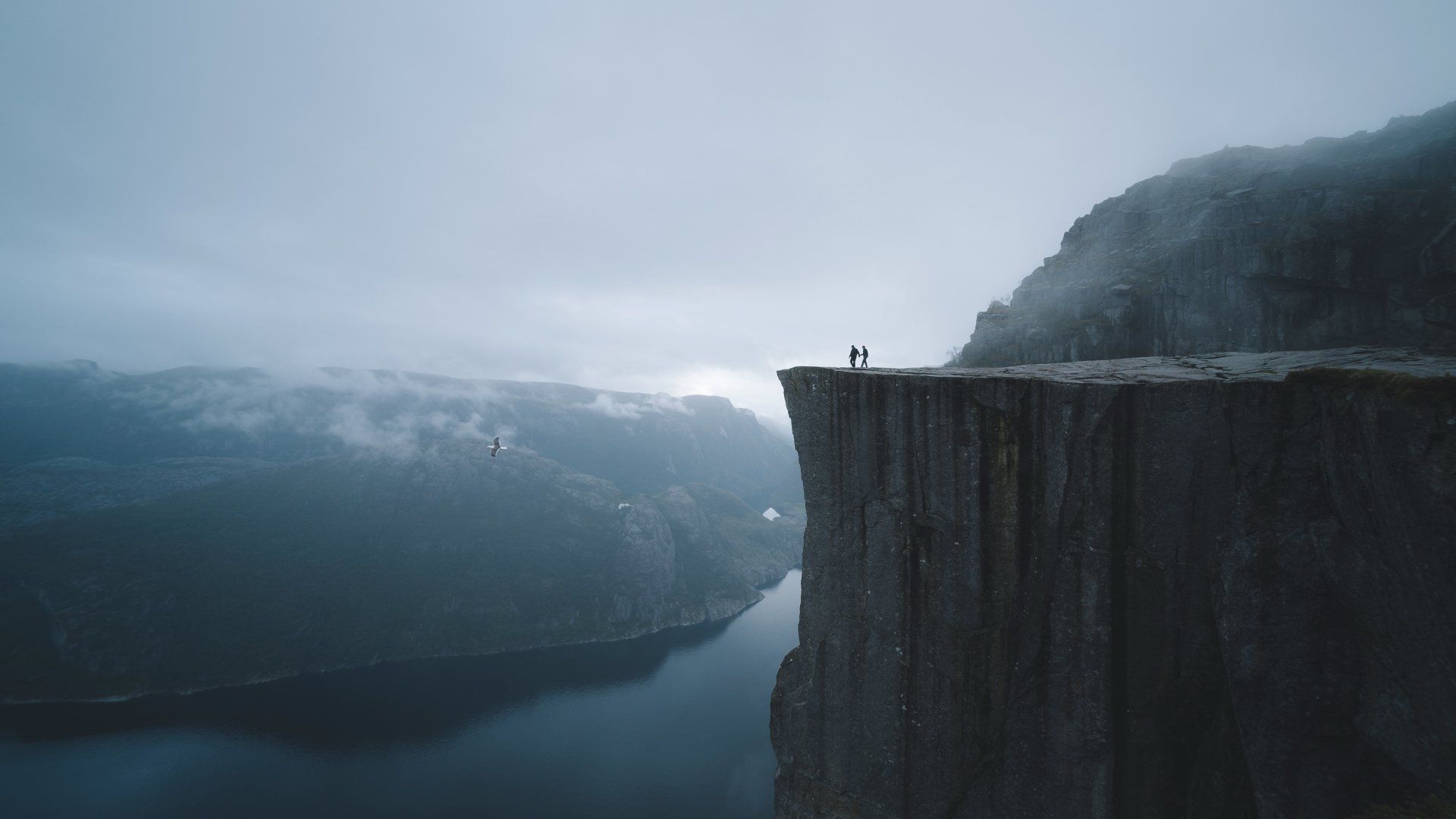
The reduction of Czech-based Notified Bodies (NBs) leaves only one still pending accreditation and one in Slovakia that does accept submissions in Czech. The delay in accreditation has produced a significant backlog for submissions pending review and acceptance. The complexity of MDR is more stringent both for the preparers and the reviewers at the NB. This situation introduces many vulnerabilities into the submission process and represents substantial risk that can and should be minimized! The potential consequences of certification delays may be critical for some manufacturers. Is the cost penalty from delay because of necessary revision or rejection worth the minor economy of an in-house effort? Get your staff an assessment from a team with proven MDR success! Contact Arete-Zoe for a courtesy review of your situation and secure assistance that will reduce your risk! Stay agile, competitive, and profitable! What is the current situation? Regulation (EU) 2017/745 on medical devices (the Medical Device Regulation, MDR), which was adopted in April 2017, became applicable in the European Union on 26 May 2021, after a year delay due to Covid [i] . The certificates issued under the existing Directives for medical devices ( 93/42/EEC and 90/385/EEC ) will expire on or before May 24th, 2024. By then, all manufacturers who wish to keep their products on the market as medical devices, will have to upgrade their documents to the new standards. Previous documentation standards fall far short to the new requirements, placing significant burden on both the manufacturers and reviewers at the NB. Many previous submissions under MDR have been rejected based on documentation shortfalls within any of the many sections. When considered with the significant backlog, any aspect of documentation that requires revision only compounds certification delay and may jeopardize market access for many medical devices. All this needs to be done within a period that accounts for the time necessary for review prior to expiration of current certifications. Additionally, there is a significant bottleneck in submission processing due to the limited capacity of NBs in the EU due to a reduction in the number of designated NBs, increased number of products that are subject to review by NB due to reclassification, and increased complexity of MDR submissions compared to MDD/AIMD. The Czech Republic still does not have its own designated NB. Once accreditation occurs, the backlog of Czech language submissions will be overwhelming. The high number of returns and requests for amendments and revisions shall be expected in the initial months, slowing the process further for all. By May 2024, many manufacturers will find themselves in a situation when their products will no longer be marketable in the EU due to expired certificates and face additional consequences from having products purged from supply chain. Discussion MDR is here to stay. Czech Minister of Health MUDr. Vlastimil Válek in his introductory statement at the October 2022 AVDZP Conference [ii] stated that another postponement of MDR is out of question. While it is not in the interest of the European Union to be dominated by non-EU manufacturers, primarily from Asia, it is unlikely that the existing Regulation will be substantially changed to accommodate manufacturers’ concerns. Válek also reminded the public that the Wild East mentality with improvised devices throughout the hospital system that dominated the Czech market in the 1990s is gone and will not return. In short, MDR is a reality to which manufacturers will have to adjust. Notified bodies available to Czech manufacturers under MDR The number of EU NBs that are designated under MDR has increased to 34, half of which are located in Italy (9) and Germany (8). The remaining 17 are in Belgium (1), Croatia (1), Finland (2), France (1), Hungary (1), Ireland (1), the Netherlands (3), Norway (1), Poland (2), Slovakia (1), Slovenia (1), Spain (1) and Sweden (1) [iii] . European manufacturers can pursue certification of their products with any EU NB, with limitations to the type of product and the capacity and willingness of NBs to take on new clients. However, for access to the greatest number of NBs, the submission should be in English. Two Czech NBs were designated in Czechia under the 93/42/EEC (MDD): Institut pro testování a certifikaci, a.s. (ITC) and Elektrotechnický Zkušební Ústav, s.p. (EZÚ). Only one of them, ITC, is in the process for designation under MDR. However, the final scope of MDR codes ITC will be able to process has not been released. One additional institution, Czech Metrological Institute (CMI) is pursuing designation under MDR without prior history in medical devices under MDD or AIMD. The anticipated accreditation will take an additional year. Institut pro testování a certifikaci, a.s. (ITC), Czech Republic ITC’s current designations include Regulation (EU) 2016/425 Personal protective equipment, 2014/68/EU Pressure equipment, 2009/48/EC Safety of toys, Regulation (EU) No 305/2011 - Construction products and 2014/30/EU Electromagnetic compatibility. Designations under 93/42/EEC Medical devices and 98/79/EC In vitro diagnostic medical devices expired in May 2021 (MDD) and May 2022 (IVDD) [iv] . ITC will continue to perform audits under MDD during the transitional period until May 24, 2024. ITC submitted their application for designation under MDR on December 30, 2019. The Designating authority (ÚNMZ) verified completeness of the application and forwarded it to the European Commission in November 2020. The Commission designated the Joint Assessment Team in December 2020, which completed the evaluated in March 2021. On-site Joint Assessment was completed in June 2021. The official JAT report was issued in September 2021. ITC implemented CAPAs in fall 2021. In June 2022, designating authority ÚNMZ completed their review of CAPAs implemented by ITC and submitted it to the Joint Assessment Team for approval. In August 2022, ÚNMZ issued the final report. In September 2022, JAT issued its final assessment and a in October 23022, ITC underwent MDCG review and is now awaiting MDCG opinion that the notification can be accepted. The expectation is that ITC should be listed in the NANDO database by January 2023 for MDR [v] . According to Mgr. Jiří Heš, ITC will primarily serve Czech manufacturers who already are their clients and have certificates issued by them under MDD. New certificates under MDR won’t be issued in time and there will inevitably be a gap in coverage of products with valid MDD certification. [vi] ITC only began preparing application for designation under IVDR. Due to the extensive backlog of MDR certificates, it is reasonable to expect that the process of designation under IVDR will not be any faster. ITC currently does not offer any training on MDR for manufacturers or guidance on how to prepare submissions. The most significant thing any manufacturer can do for themselves is to ensure their submission is as appropriate as possible the first time. But they are left to their own means to sort out the transition from MDD to MDR. Without specific guidance from ITC on their expectations, there is significant risk from the ambiguity of the MDR Regulation itself. However, this risk can be reduced from experience from submissions under MDR to other NBs which will provide reasonable opportunity for a successful submission. Elektrotechnický Zkušební Ústav, s.p. (EZÚ), Czech Republic EZÚ’s current designations include Regulation (EU) No 305/2011 - Construction products and 2014/30/EU Electromagnetic compatibility. EZÚ won’t pursue designation under MDR. The activities EZÚ will continue include audit under MDD during the transitional period for 42 manufacturers whose certificates they serve, certification of quality management systems, and electrical safety. EZÚ also provides training on MDR for manufacturers and distributors [vii] . Czech Metrological Institute (CMI), Czech Republic CMI submitted their initial application in December 2020, a year later than ITC. The Joint Assessment Team evaluation (Article 39, paragraph 4 MDR) was completed in December 2021. In May 2022, CMI submitted their proposed CAPA plan. CMI strives to maintain the scope of devices included in their application (23 out of 44 basic codes and 18 out of 27 horizontal codes). The key requirement is to prove personnel availability for each code. CMI primarily intends to serve Czech manufacturers in Czech language, as only about a third of their reviewers have sufficient proficiency in English. CMI does not provide any training or guidance for manufacturers how to prepare documentation to pass their scrutiny. Certification of products on the Czech market There are over 400 Czech manufacturers listed in the database RZPRO holding nearly 6000 certificates that are currently valid. Of these, 4323 did not previously require the involvement of a NB (Class I). However, due to reclassification, some of these will require involvement of the NB under MDR, further stressing the throughput of the NB. Of the 1634 remaining certificates, 514 were issued by EZÚ and 391 by ITC. Another 133 MDD certificates were issued by other NBs that do not currently have MDR designation. The database also holds 2305 products other than Class I that do not have a NB listed. In total, ITC issued 541 certificates that are currently valid, including manufacturers from other countries. The database currently holds 630 valid certificates issued by EZÚ. All products certified by EZÚ will have to be recertified by another NB [viii] . The list of MDR codes that will be covered by ITC (or CMI, once designated) is not currently available. Mgr. Irena Storova (Czech State Institute for Drug Control) emphasized the quality of the MDR documentation as an essential condition required to avert a crisis. The current speed of issuing certificates by EU NBs is about 1.000 certificates a year. However, the anticipated need for medical devices in Europe is about 23.000 certificates issued by NBs over the period of 20 months, making the transition to MDR extremely challenging. The key problems are insufficient capacity of NB, partly due to accumulated backlog, partly due to increased complexity of MDR compared to MDD/AIMD, as well as inadequate preparedness of manufacturers to meet the new complex requirements of MDR [ix] . Experience of other EU NB shows exponential growth of applications and a serious lag in processing certificates. In February 2021, NBs received 1840 applications and processed 224 certificates. By October 2022, the number of applications grew to 8120, while the number of issued certificates was 1990 [x] . These numbers suggest further accumulation of backlog of unprocessed applications rather than catching up with the growing demand. The time to reach a Certificate according to MDR (MDR Quality Management System and Product certification) typically ranges from 13 to 18 months [xi] . This is consistent with ITC’s estimate that the review of a complete submission will take a year to issue a certificate. This means that all manufacturers who need their certificates renewed before the May 2024 deadline when they all expire will have to submit their applications before May 2023. Since ITC will only start accepting applications after the official MDR designation, it is safe to assume that all applications will be submitted in the period between the designation date and May 2023, creating a long backlog queue. To complicate the situation even further, manufacturers, whose MDR codes won’t be on ITC’s list, will have to look for a different NB to pursue their certificates. Czechia as a low price-point market The low-price level of devices on the Czech market complicates the transition to MDR even further. The system of defining reimbursements for medical devices is very rigid, and there are multiple pressures that keep prices down. These price controls conflict with increased costs associated with certification and recertification of products when considered with increased costs of energy, raw materials, transport, and labor. MUDr. Vlastimil Válek at the AVDZP Conference on October 13, 2022, stated that it is not in the interest of the European Union to allow non-EU manufacturers to dominate the EU market, and that there is an intrinsic value in self-sufficiency in times of crisis [xii] . The results from Survey on certifications and applications performed by MDCG & Stakeholders among NBs (51 NBs asked, 47 replies received) suggest that 60% of medical device clients are non-EU (10,913), compared to 7,143 EU clients [xiii] . Priority treatment for small business is not likely since SMEs are the majority of NB clients, both EU-wide and locally in the Czech Republic. In fact, as the threat for shortage of medical equipment becomes more urgent, policymakers might solve the crisis by prioritizing producers with high capacity, capable of meeting the demands of their respective health systems, as we have seen with the imports of medical equipment and protective materials during the Covid crisis. What is the risk to business due to delays in certification? The ability of NBs to process applications in a timely manner is an essential condition for the function of the medical device sector. This is directly influenced by the quality of manufacturers’ submission for certification. Manufacturers cannot legally keep their products on the EU market without valid certification. And, additional expenses will be incurred, if products have to be recovered from distribution chains due to delays in certification. Furthermore, this will create gaps in coverage, creating a void that can be filled by competitors. Extended periods of absence can stall otherwise good products and limit marketability once certification is finally obtained. Failure to obtain MDR certification for any portion of a product portfolio is an existential threat to any enterprise, potentially forcing reduction of staff, downsizing, making the enterprise vulnerable to competitors, or simply losing viability and going out of business. The absence of a feedback loop regarding the minutia of submissions’ content and format will cause additional delays and adjustments by the industry. ITC does not provide any MDR training and did not issue any guidance documents to facilitate successful submissions and reduce ambiguity relating to the novelty of the process. The first MDR certificate was issued in September 2019 by BSI [xiv] . This means that the cumulative experience in the industry among competition is significantly higher, placing Czech manufacturers 4 years behind other EU players. At present, Czech manufacturers have to rely on training provided by other NBs, industry consultants who rely on the same, in addition to feedback from their clients, and their own understanding of the Regulation and associated MDCG guidelines. Although CzechInvest does have a plan to start a comprehensive training program for manufacturers to facilitate the transition to MDR, the course has not started yet. The first students are expected to graduate in June 2024 [xv] . Learning curve by both newly designated NBs as well as manufacturers will inevitably affect the speed of processing, forcing reworks and amendments that would otherwise be avoidable. Securing consultancy with demonstrated MDR competency will remove substantial risk from the current situation. Recommendations What is the risk, and how does Arete-Zoe help your enterprise to mitigate such risk? The key to a successful MDR certification is a high quality, timely submission that complies with or better, exceeds minimum MDR requirements, ensuring timely processing and avoiding returns, requests for more information and outright rejections. It is important to note that the review and approval process depends on the understanding and application of MDR requirements by individual reviewers. Therefore, exceeding minimum requirements becomes a necessity for confident approval. The impact of additional information requests, although minor in terms of extra work, can be significant due to delays. Additionally, other manufacturers’ failed submissions in a long backlog of applications will continue to burden the system. The quality of the initial submission is essential to avoiding consequences in the transition to MDR and may be the single factor that keeps the business open. Manufacturers who have a fully trained in-house team already should still expect challenges in preparing MDR submissions themselves. The time to develop MDR expertise is very limited, considering the pressure to prepare the full documentation and submit it before May 2023. Even with a well-staffed team, the task can simply be overwhelming due to the sheer volume of material required in contrast to previous MDD/AIMD submissions. Arete-Zoe team has significant experience preparing clinical documentation for clients transitioning from MDD to MDR in both Czech and English and submissions through multiple NBs. Our exceptional success record with clinical documentation includes products that were previously rejected but passed with our assistance. We can help control the risk of failure or delay for our clients by providing the essential support you need to avert a avoidable delays in product certification. Arete-Zoe team can either prepare the full submission or augment your existing team with essential skillset your team might not have. References [i] https://www.ema.europa.eu/en/news/medical-device-regulation-comes-application [ii] Válek, V (2022). Introduction. AVDZP Conference 13/10/2022, Praha, Czech Republic. [iii] NANDO database https://ec.europa.eu/growth/tools-databases/nando/index.cfm?fuseaction=directive.notifiedbody&dir_id=34 [iv] NANDO database https://ec.europa.eu/growth/tools-databases/nando/index.cfm?fuseaction=directive.notifiedbody&dir_id=34 [v] Heš, J (2022). Implementace MDR: Kde jsme a kam směřujeme. AVDZP Conference 13/10/2022, Praha, Czech Republic. Institut pro Testování a Certifikaci. [vi] Heš, J (2022). Implementace MDR: Kde jsme a kam směřujeme. AVDZP Conference 13/10/2022, Praha, Czech Republic. Institut pro Testování a Certifikaci. [vii] Vlasák, M (2022). Dozorová činnost dle MDD. AVDZP Conference 13/10/2022, Praha, Czech Republic. Elektrotechnický zkušební ústav. [viii] Czech database of medical devices RZPRO https://eregpublicsecure.ksrzis.cz/Registr/RZPRO/ZdravotnickyProstredek [ix] Storova, I (2022). Problematika ukončení přechodného období MDR z pohledu SÚKL. AVDZP Conference 13/10/2022, Praha, Czech Republic. State Institute for Drug Control. [x] MDCG & Stakeholders (2022). Notified Bodies Survey on certyifications and applications (MDR/IVDR). 24/10/2022. European Commission. [xi] MDCG & Stakeholders (2022). Notified Bodies Survey on certyifications and applications (MDR/IVDR). 24/10/2022. European Commission. [xii] Válek, V (2022). Introduction. AVDZP Conference 13/10/2022, Praha, Czech Republic. [xiii] MDCG & Stakeholders (2022). Notified Bodies Survey on certifications and applications (MDR/IVDR). 24/10/2022. European Commission. https://health.ec.europa.eu/latest-updates/notified-bodies-survey-certifications-and-applications-2022-10-26_en?fbclid=IwAR3w3YH7UD2HBccQ6pBKWP3UlpgSnvQj9qFoNUeLIF-6ZWl8IOwP2Wx88Tk [xiv] BSI (2019). BSI certifies first product to the Medical Devices Regulation. 02 September 2019. BSI. https://www.bsigroup.com/en-GB/medical-devices/news-centre/enews/2019-news/bsi-certifies-first-product-to-the-medical-devices-regulation/ [xv] Hájek, J (2022). Národní plán obnovy. Komponenta 1. 4. Digitální ekonomika a společnost, inovativní start-upy a nové technologie. Program na podporu specifických systémových a produktových certifikací a souvisejícího vzdělávání. AVDZP Conference 13/10/2022, Praha, Czech Republic.
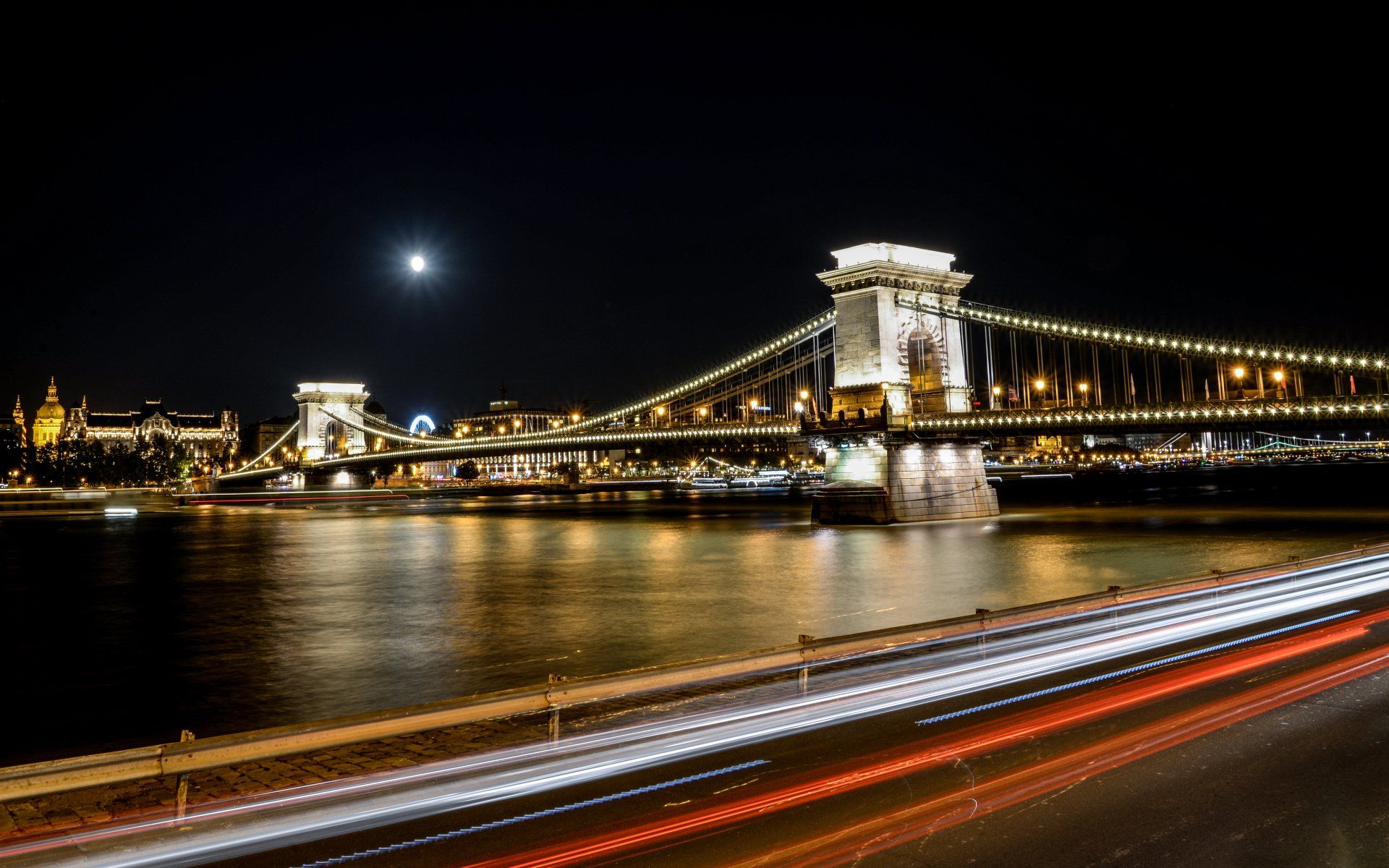
More than 500,000 medical technologies are available on the European market, from hospitals to community care settings and people's homes. The products range from syringes, pregnancy tests, and wheelchairs to X-Ray machines and body scanners, pacemakers, hip implants, and pharmacogenomic tests. The medical technology industry is the source of a constant flow of innovations. The sector spends about 8% of its sales on R&D. Typical product lifecycle is about 18 to 24 months when a new, improved version becomes available. In 2020, the European Patent Office (EPO) accepted nearly 14,200 patent applications in the medical technology sector, trumping pharmaceutical patents (8,500 applications) and biotechnology (7,200). European and U.S. entities filed almost 80% of the applications (38% EU and EEA, 39% U.S.) [ 1 ]. The European medical technology sector employs more than 760,000 people, mainly in Germany (210,000), the United Kingdom (102,800), Italy (94,000), France (89,000), Switzerland (63,000), and Ireland (40,000), accounting for 0.3% of total employment. In comparison, the European pharmaceutical industry employs around 795,000 people. These jobs reach a value-added of about €184,000 per employee. More than 33,000 medical technology exist in Europe, of which 95% qualify as small, medium, and micro-sized companies (SMEs). The majority of these enterprises employ less than 50 people [ 1 ]. In 2020, Europe had a positive trade balance in the medical technology sector of € 8.7 billion. Compared to 2019, the European trade balance dropped by 27.5% (€ 12 billion in 2019). The most important trading partners for Europe are the United States, China, Japan, and Mexico. Germany, Ireland, the Netherlands, Belgium, and Switzerland have the highest trade share, both within and outside the EU [ 2 ]. Until May 2021, the medical device sector was regulated by Medical Device and In Vitro Diagnostic Device Directives 93/42/EC and 90/385/EEC (MDD and IVDD), when the new Regulations replaced these: Medical Device Regulation (EU) 2017/745 (MDR) and In Vitro Diagnostic Device Regulation (IVDR) 2017/746 [ 3 ],[ 4 ]. The new regulations introduced numerous changes, including the reclassification of some devices, requiring additional obligations for manufacturers to comply with. About 85% of in-vitro diagnostic devices will now require Notified Body involvement, compared to ~20% under the IVDD. Existing MDD/IVDD certificates remain valid until May 2024. After this date, all devices on the EU market must comply with the new MDR/IVDR regulations [ 5 ]. For some manufacturers, the costs associated with keeping some of their devices on the market under MDR/IVDR may no longer justify the expense considering their profitability. Others may not be able to obtain new CE Mark certification in time due to decreased capacity of notified bodies. These factors combined are already reducing the number of devices on the EU market and limiting the certification of innovative products. The number of notified bodies available to review new certifications and recertifications dropped significantly under the MDR/IVDR. Of 51 notified bodies designated to MDD [ 6 ] and ten to AIMDD [ 7 ], only 29 obtained designation for MDR, of which seven operate in Germany, seven in Italy, and three in the Netherlands [ 8 ].
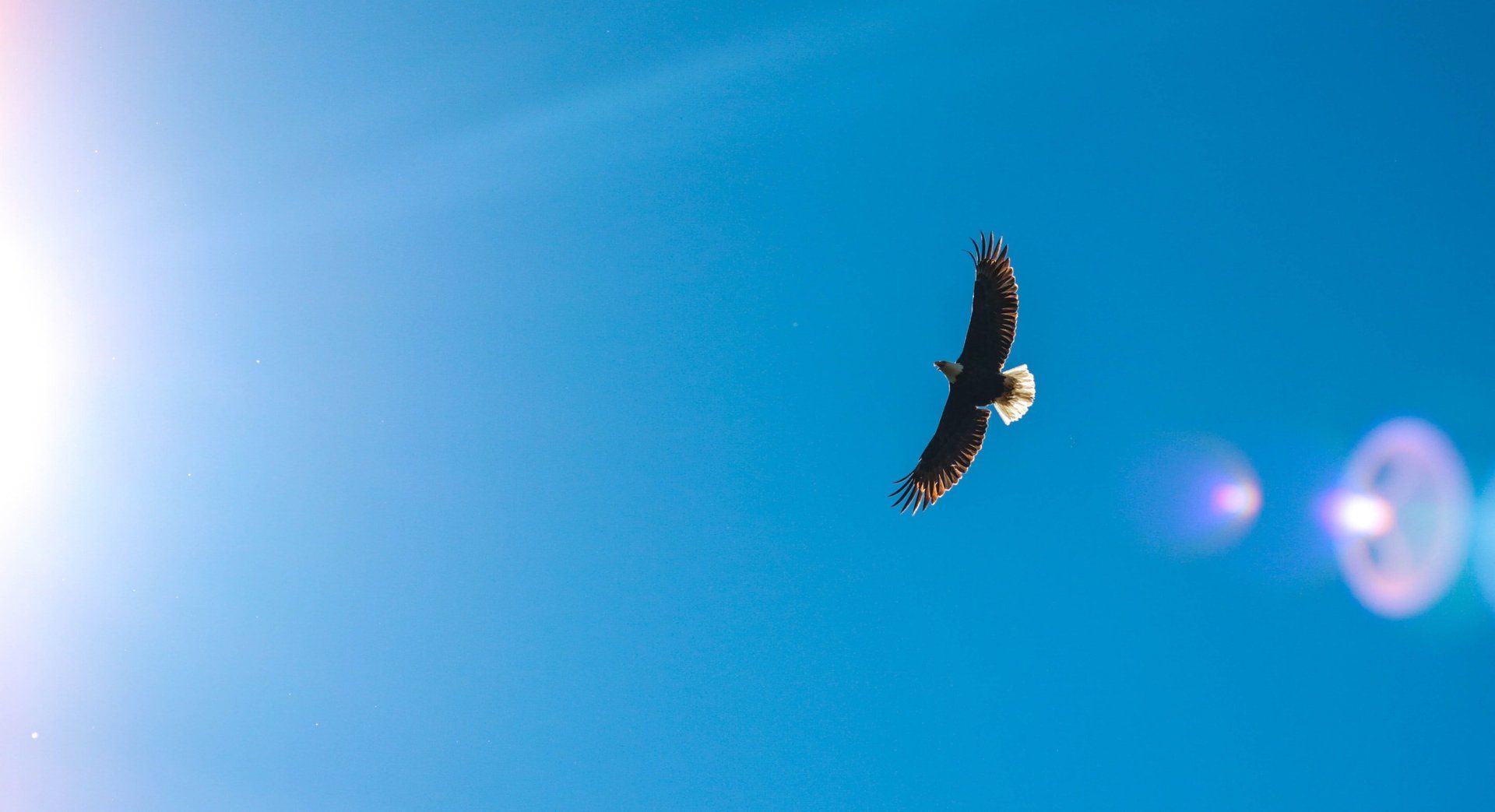
The Medical Device industry produces a vast number of products, ranging from bandages and surgical instruments to life function monitors to imaging technology. The technology currently in use varies from devices that have been in use for decades to highly innovative products. Innovations in the medical device field are frequent and typically incremental in response to feedback from physicians. Mordor Intelligence estimated the global Medical Devices market at USD 532.62 billion in 2021, growing at a CAGR of around 5.5%, to reach USD 734.39 billion in 2027 [1]. Fortune Business Insights projects the global medical devices market growth from $455.34 billion in 2021 to $657.98 billion in 2028 at a CAGR of 5.4%. This development follows a decline from 2020, when CAGR dropped to 3.7% due to the pandemic [2]. The key drivers of market growth include the rising prevalence of chronic diseases, increased disability throughout the population, technological advancements in medical devices, and population aging. The U.S. medical device market was valued at USD 186.5 billion in 2021 and is anticipated to exhibit a compound annual growth rate (CAGR) of 5.0% over the forecast period to reach USD 262.4 billion in 2028 [4]. The Medical Device sector is even larger than Biopharmaceuticals, that by comparison, employed over 224,000 people, earning $21.2 billion [3]. The rising prevalence of chronic diseases and the increasing geriatric population in the country are the key market drivers. The Medical Device industry has a significant impact on the U.S. economy and supports hundreds of thousands of jobs. More than 80 percent of U.S. medical device companies have fewer than 100 employees [5]. In 2020, the U.S. Medical Device industry supported over 329,000 jobs, with an annual payroll of $25.8 billion [3]. Employment and payroll for Medical Device Subsectors, 2020 ( SelectUSA ):
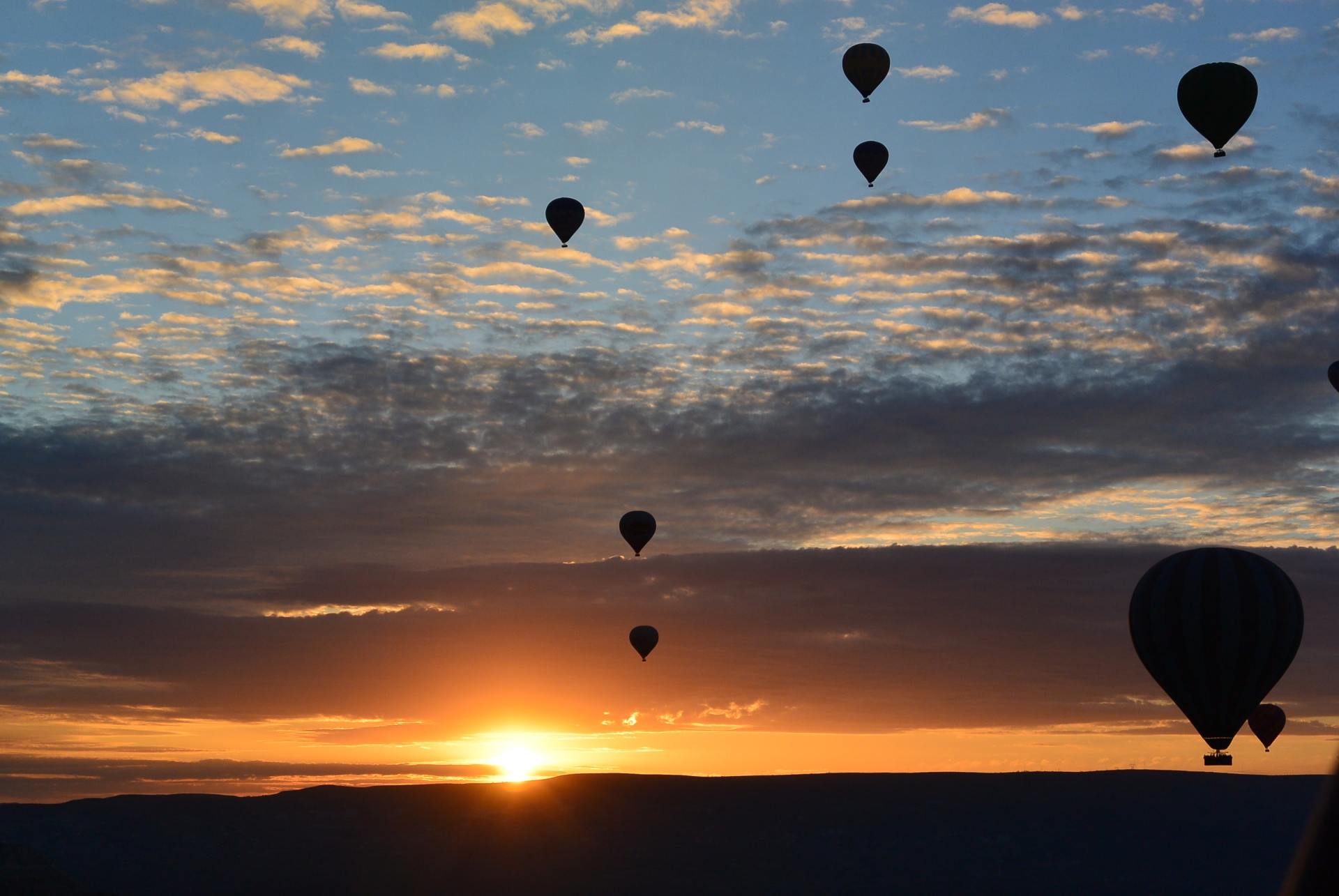
In the high consequence environment of pharmaceutical development, any assumption made earlier in the process can prove extremely costly if uncorrected once more information becomes available. From a business perspective, it is essential to create a safe avenue for communication of concerns regarding the drug candidate’s efficacy, safety, toxicity, or pharmacological function immediately as the researchers become aware of them.
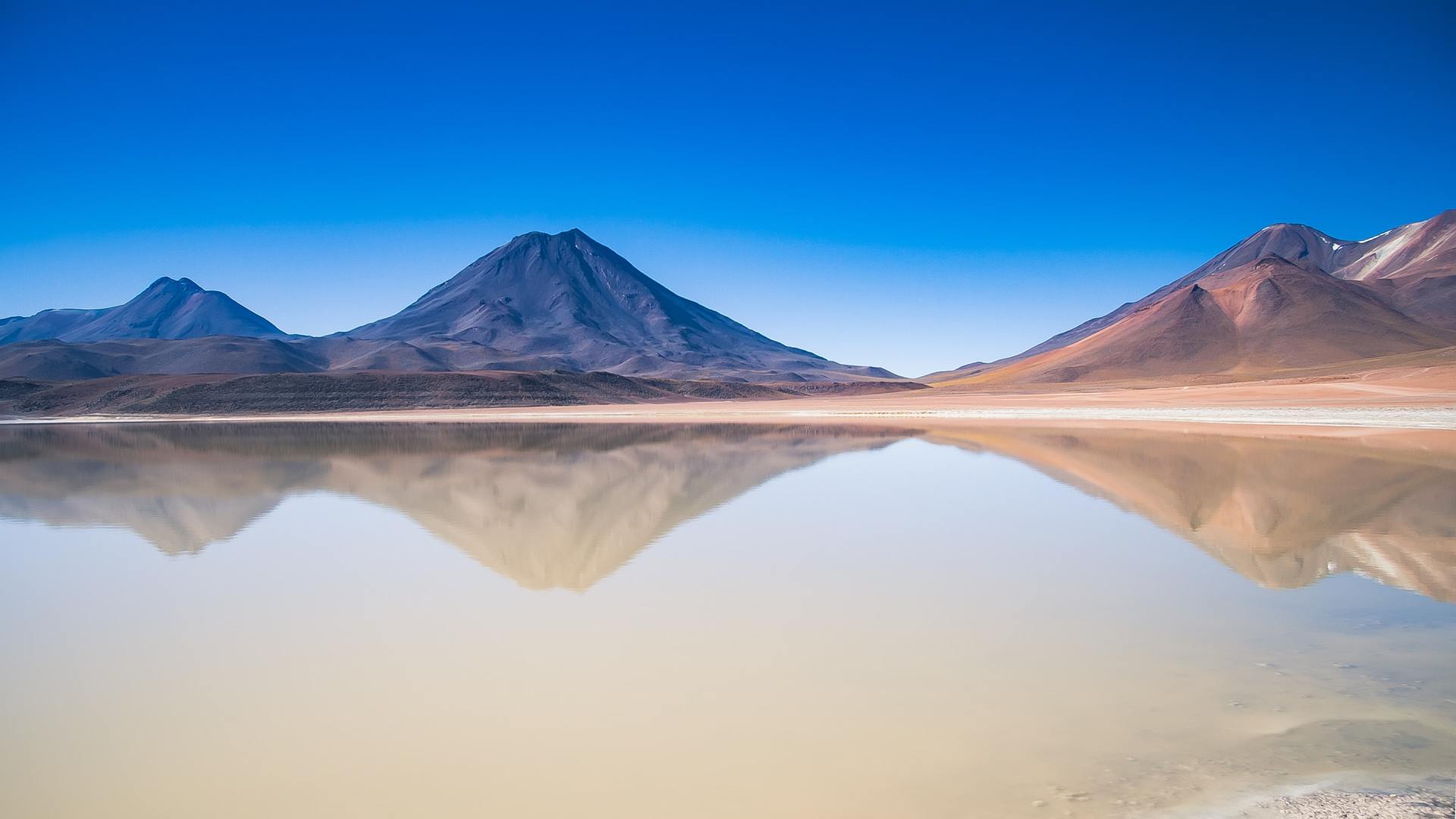
Innovation always involves the risk of failure. It is an art to see what the data show, and what they don't, and which projections are the result of our wishful thinking or unsubstantiated assumptions. It may be just my impression that 14th-century logician William of Ockham whispers in my ear that entities shall not be multiplied unnecessarily.
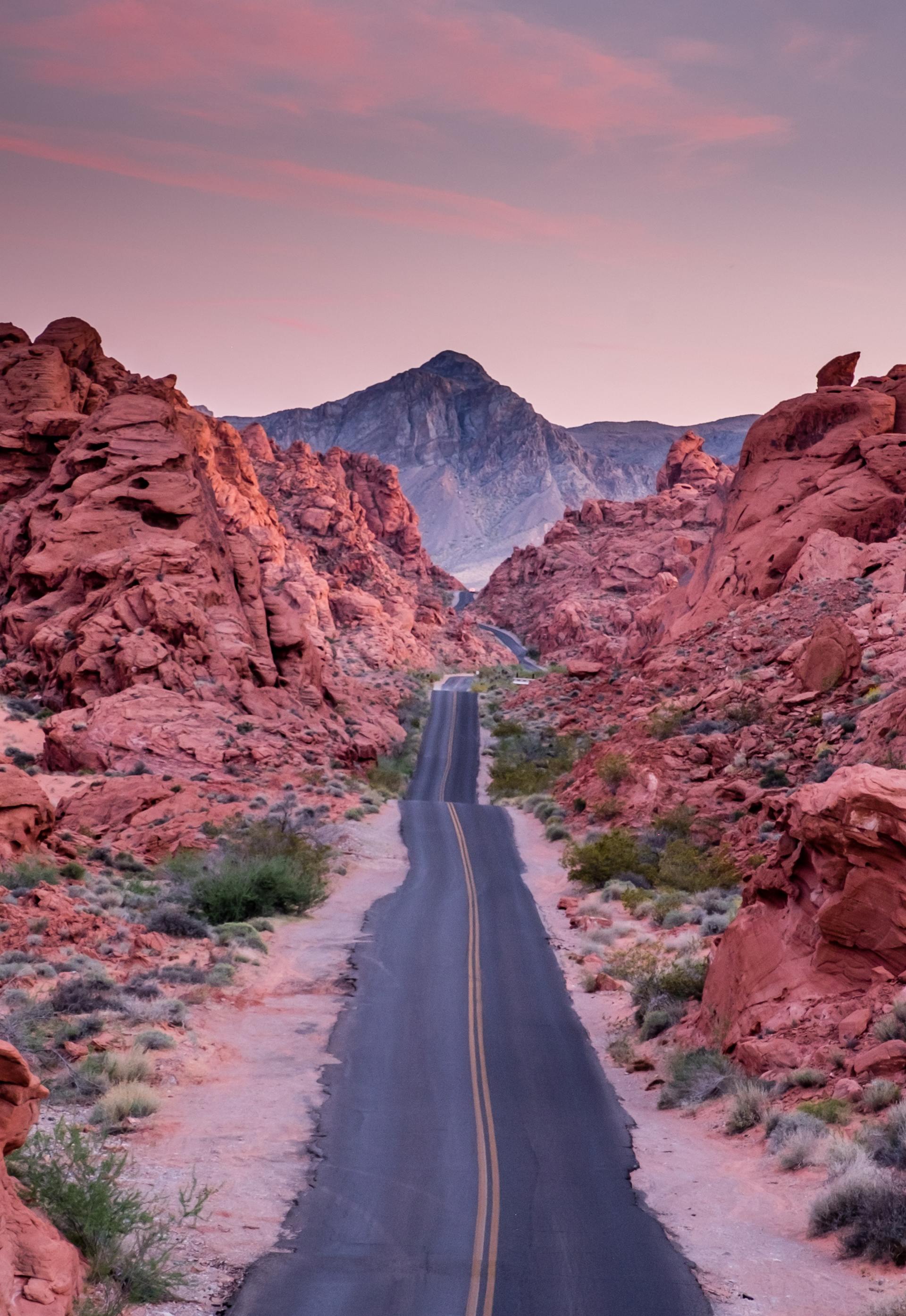
Companies are growing in size due to acquisitions and mergers. Operations routinely span across geographical, jurisdictional, and cultural boundaries. The trend of industry consolidation continues in 2015 and 2016: the total number of deals flattened and remained even at around 600 mergers a year. Geographically, mergers, and acquisitions have been shifting from the U.S. to Western Europe. This shift is the result of transactions driven by the need to add complementary products to the core business areas and tax inversions.